Stars are amazing. Lots of things depend on them. So, figuratively speaking, they are your local centers of the universe.
A star is a massive, luminous, self-gravitating ball of plasma. And the force that triggers a stellar timer is gravity. So, once it is on, things start to happen. During its lifetime a star undergoes a sequence of changes. These changes affect everything in the vicinity of a star; and maybe a little further than that. Stellar evolution is studied by observing numerous stars at the various points of their life, and by simulating stellar structure with computer models.
Before choosing a star (or a multiple star) for your system, you might consider some options here: life as we know it; life as we don’t know it, or no life at all. And if the latter, what next? Will there be someone arriving or is it just a dead world?
In this article I will stick to the life as we know it scenario.
This leaves me with a limited choice of suitable stellar types from the main sequence range between a late F and an early/mid K. Because these are the ones that are more or less suitable having an earthlike world (be it a planet or a moon) in terms of habitability according to the UV constrains and other accepted criteria of habitability (liquid water on planet’s surface, comparable surface inventories of carbon dioxide, water, dinitrogen and other biogenic elements, an early history allowing chemical evolution that leads to life, and subsequent climatic stability for several billions of years).
Stars earlier than F0 have very short (2 Gyr) main sequence lifetimes and, hence, have a low probability of harboring planets with complex life. Their habitable zones migrate outwards rapidly, so even microbial life might not have time to develop in these systems. Planets orbiting stars later than K5 are likely to become tidally locked, which may be a problem for habitability. Or maybe not, but it is a different case, though no less interesting.
Main sequence stars of spectral class F8V to K2V (or 0.50 to 1.00 in B−V) are similar to the Sun in mass and evolutionary state, and are usually called solar-like stars.
Circumstellar habitable zones
The habitable zones of main sequence stars have traditionally been defined as the range of orbits that intercept the appropriate amount of stellar flux to permit surface water on a planet. The inner HZ boundary is determined by the loss of water via photolysis and hydrogen escape. The outer HZ boundary is determined by the condensation of carbon dioxide crystals out of the atmosphere.
However, in the case of life as we know it, this is not the only factor to consider.
Ultraviolet radiation is known to inhibit photosynthesis, induce DNA destruction and cause damage to a wide variety of proteins and lipids. In particular, UV radiation between 200-300 nm becomes energetically very damaging to most of the terrestrial biological systems. On the other hand, UV radiation is usually one of the most important energy sources on the primitive Earth for the synthesis of many biochemical compounds and, therefore, essential for several biogenesis processes (Buccino et al. 2006, 2007).
Chromospheric UV radiation is increased in young stars in regard to all stellar spectral types. Compelling observational evidence (Gudel et al. 1997) shows that zero-age main sequence (ZAMS) solar-type stars rotate over 10 times faster than today’s Sun. As a consequence of this, young solar-type stars, including the young Sun, have vigorous magnetic dynamos and correspondingly strong high energy emissions.
Flare activity (an important agent for the delivery of highly energetic radiation, especially UVC) is most pronounced in K and M-type stars, which also has the potential of stripping the planetary atmospheres of close-in planets, including planets located in the stellar habitable zone. The amount of chromospheric radiation is, in a statistical sense, directly coupled to the stellar age as well as the presence of significant stellar magnetic fields and dynamo activity.
Studies have shown that chromospherically induced biological damage for planets hosted by F and G-type stars will be insignificant. The situation is, however, decisively different for K and M dwarfs. Any of these stars, including inactive stars, show noticeable chromospheric emission. For stars with basal chromospheric emission, chromosphere induced biological damage only occurs for stars of spectral type mid-K and later, whereas for stars with relatively high chromospheric emission, chromosphere-induced biological damage can also be found in the environments of very late G-type and early K-type stars (Cuntz et al., 2010).
However, photochemical/radiative–convective modeling shows that planets around K2V and F2V stars both exhibit better UV protection than does Earth. See “Ozone concentrations and ultraviolet fluxes on Earth-Like planets around other stars”, Segura et al, Astrobiology, Volume 3, Number 4, 2003.
So, in order to set your world in a right place of your solar system, you need to consider two factors – liquid water HZ and UV HZ.
Both are important factors to determine the location and the size of circumstellar habitable zone.
For life systems to evolve, the planet should be in the habitable zone continuously during a certain time. A common choice for this time is 4 Gyr, the time that was needed on Earth for intelligent life to emerge and evolve into a technological civilization. Henry et al. (1995) and Turnbull and Tarter (2003a) considered 3 Gyr, while Schopf (1993) used the time required for microbial life to emerge 1 Gyr in his definition of the CHZ.
Because main sequence stars become brighter as they age and convert hydrogen into helium and heavier elements, habitable zones tend to migrate outward with time. In order to analyze the evolution of the habitable zones, it is assumed that the UV radiation follows the same pattern as the change in luminosities of F, G and K main sequence stars.
Until an atmospheric protection is built, a planetary surface would be exposed to larger amounts of UV radiation, which could act as one of the main source in the synthesis of bioproducts and, in a certain wavelength, could be damaging for DNA. Both concepts represent the UV boundaries for the UV habzone. To obtain the exact AUs of this “safe circle”, you might consider using formulas for UV habitable zones around host stars presented by Guo et al, 2010.
For traditional luminosity-water habitable zone calculations see Kasting et al.,1993.

The traditional and the UV HZ must at least partially overlap for a considerable amount of time in order to host a habitable planet in it.
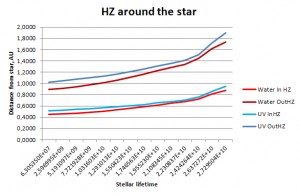
Things you’ll need to get started
As I mentioned before, stars evolve. You need evolutionary tracks of the star if you are interested in the long term evolutionary path of your world and its inhabitants, whatever those might be.
First of all, decide on a mass and effective temperature of your star. Those usually correspond to the spectral class (F8V…K2V or later). You might actually want to use an existing star from a catalogue to get all the parameters necessary. And don’t forget to look up star’s actual age. It will be your anchor.
In order to obtain evolutionary tracks of any star you might choose, you don’t have to derive any formulas. It has already been done. You simply can use stellar model grids. Personally, I like to use Stellar models grids I. Z=0.02, M=0.8 to 125 Msol by Claret, 2004, which present stellar models suitable for the mean solar neighborhood, i.e. for Z=0.02 (metallicity) and X=0.70 (hydrogen). The covered mass range is from 0.8 up to 125Msol and the models are followed until the exhaustion of carbon in the core, for the more massive ones. In addition, the effective temperatures of the more massive models are corrected for the effects of stellar winds, while models with lower effective temperatures are computed using a special treatment of the equation of state. You can read a more detailed description here and see the grids here.
The grid model has time steps in years and you can compare it with your selected star’s data (i.e. in luminosity, effective temperature and other things according to age, which is your anchor).
Try to experiment with different grids for other element abundance ratios. They can be found here. (E.g. Grids of stellar models. VIII. From 0.4 to 1.0 Msun at Z=0.020 and Z=0.001, Charbonnel+ 1999, description).
When you’re done, you’ll have a full table of stellar lifetime parameters, which later can be used as a basis for all of your calculations. These include mass, luminosity, effective temperature, central density and temperature, element abundancies and some other stuff like fractional gyration radius at several age steps.
Have fun, and more exciting stuff to follow.