This is going to be a brief introduction to the climate modeling beyond those atmospheric models I did earlier. Global-scale climate models, which simulate the climate of the entire planet, are of a particular interest here.
Climate is the long-term statistical expression of short-term weather. It varies over different time scales ranging from years to hundreds of millions of years, and each periodicity is a manifestation of separate forcing mechanisms. The overall state of the global climate is determined by the balance of solar and terrestrial radiation budgets.
When constructing or selecting a climate model, one must consider three groups of processes: the transfer of radiation through the climate system (e.g. absorption, reflection); the horizontal and vertical transfer of energy (e.g. advection, convection, diffusion); and the inclusion of processes involving land/ocean/ice, and the effects of albedo, emissivity and surface-atmosphere energy exchanges. And, of course, due the limitations imposed by incomplete understanding of the climate system and computational constraints, climate models are usually simplified versions of this very complex climate system.
There are four main categories of climate models: energy balance models (EBMs); one dimensional radiative-convective models (RCMs); two-dimensional statistical-dynamical models (SDMs); three-dimensional general circulation models (GCMs). The simplest models permit little interaction between the primary processes, radiation, dynamics and surface processes, whereas the most complex models are fully interactive.
Computational cost is always an important factor to consider when choosing a climate model, and certain processes may be omitted from the model if their contribution is negligible on the time scale of interest.
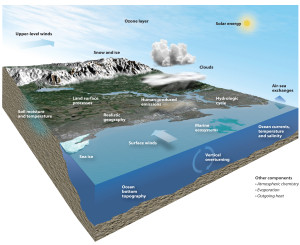
The Big Five of the Global Climate System
The global climate system is a consequence of and a link between the atmosphere, oceans, the ice sheets (cryosphere), living organisms (biosphere) and the soils, sediments and rocks (geosphere). In this post the Earth’s climate system is discussed. On extrasolar planets with different obliquities, insolation rates, and other elements not like of the Earth this whole system will be quite different story (I will speak about it in details in future posts.)
The Atmosphere
The atmosphere is a mixture of gases and aerosols that forms an integrated environmental system with all the Earth’s components. It is not physically uniform and has significant variations in temperature and pressure with altitude. The atmosphere does not respond as an isolated system. It influences the energy budget of the global climate system and the energy transfers of the Earth-atmosphere system are in equilibrium. The fluxes of energy, moisture momentum and mass determine the state of the climate.
The Oceans
The thermodynamic state of the oceans, like the atmosphere, is determined by the transfer of heat, momentum and moisture to and from the atmosphere. These fluxes within this coupled ocean-atmosphere system also exist in equilibrium (the other components of the climate system are ignored here.)
Surface winds transfer momentum is transferred to the oceans, mobilizing the global surface ocean currents. Surface ocean currents assist in the latitudinal transfer of heat similar to the process occurring in the atmosphere. Warm water moves towards the poles whilst cold water returns towards the equator. Moisture is another mechanism to transfer energy. Water evaporating from the surface of the oceans stores latent heat which is subsequently released when the vapor condenses to form clouds and precipitation.
Ocean stores much greater quantity of energy than the atmosphere because of its larger heat capacity (4.2 times that of the atmosphere) and its much greater density (1000 times that of air). The vertical structure of the ocean can be divided into two layers which differ in the scale of their interaction with the overlying atmosphere. The lower layer comprises the cold deep water sphere, making up 80% of the oceans’ volume. The upper layer, which has closest contact with the atmosphere, is the seasonal boundary layer, a mixed water sphere extending down only 100 m in the tropics but several kilometers in polar regions. The seasonal boundary layer alone stores approximately 30 times as much heat as the atmosphere (Henderson-Sellers & Robinson, 1986). Thus for a given change in heat content of the ocean-atmosphere system, the temperature change in the atmosphere will be around 30 times greater than that in the ocean. Therefore small changes to the energy content of the oceans could have considerable effects on global climate.
Energy exchanges also occur vertically within the oceans, between the mixed boundary layer and the deep water sphere. Sea salt remains in the water during the formation of sea ice in the polar regions, with the effect of increased salinity of the ocean. This cold, saline water is particularly dense and sinks, transporting with it a considerable quantity of energy. To maintain the equilibrium of water (mass) fluxes, a global thermohaline circulation exists, which plays an important role in the regulation of the global climate. Broecker & Denton (1990) have proposed that changes in this thermohaline circulation influence climate changes over millennia time scales.
Additional reading: The Thermohaline Ocean Circulation
The Cryosphere
These are the regions of the planet, both land and sea, covered by snow and ice, including high mountain ranges throughout the world, where sub-zero temperatures persist throughout the year. Without the cryosphere, the global albedo would be considerably lower. More energy would be absorbed at the Earth’s surface rather than reflected, and consequently the temperature of the atmosphere would be higher.
The cryosphere also acts to decouple the atmosphere and oceans, reducing the transfer of moisture and momentum, stabilizing the energy transfers within the atmosphere (Henderson-Sellers & Robinson, 1986). And as stated in the Oceans section, the formation of sea ice in polar regions can initiate global thermohaline circulation patterns in the oceans.
The presence of the cryosphere itself affects the volume of the oceans and global sea levels, changes to which can affect the energy budget of the climate system.
The Biosphere
The biosphere, both on land and in the oceans, affects the albedo of the Earth’s surface. Large areas of continental forest have relatively low albedos compared to barren regions such as deserts. The presence of the continental forests influence the energy budget of the climate system.
The biosphere also influences the fluxes of certain greenhouse gases such as carbon dioxide and methane. The oceans effectively absorb the gas from the atmosphere creating the flux of carbon dioxide. Plankton in the surface oceans utilizes the dissolved carbon dioxide for photosynthesis. On death, the plankton sink, transporting the carbon dioxide to the deep ocean. Such primary productivity reduces the atmospheric concentration of CO2 by at least four-fold, weakening significantly the Earth’s natural greenhouse effect.
The biosphere also influences the amount of aerosols in the atmosphere. Millions of spores, viruses, bacteria, pollen and other minute organic species are transported into the atmosphere by winds, where they can scatter incoming solar radiation, and so influence the global energy budget.
Primary productivity in the oceans results in the emission of compounds known as dimethyl sulphides (DMSs). In the marine atmosphere those are oxidized to various sulfur-containing compounds–sulphate aerosols called marine non-sea-salt (nss) sulphate (Charlson et al., 1987). These nss sulphates act as condensation nuclei for water vapour in the atmosphere, thus allowing the formation of clouds. Clouds have a highly complex effect on the energy budget of the climate system. Thus changes in primary productivity in the oceans can affect, indirectly, the global climate system.
Besides these major influences there are, of course, many other mechanisms and processes which couple the biosphere with the rest of the climate system.
The Geosphere
The soils, the sediments and rocks of the Earth’s land masses, the continental and oceanic crust and ultimately the interior of the Earth itself is another component of the global climate system.
Long-scale changes in the shape of ocean basins and the size of continental mountain chains (driven by plate tectonic processes) may influence the energy transfers within and between the coupled components of the climate system.
On much shorter time scales physical and chemical processes affect certain characteristics of the soil, such as moisture availability and water run-off, and the fluxes of greenhouse gases and aerosols into the atmosphere and oceans (Cubasch & Cess, 1990; McBean & McCarthy, 1990). Volcanism, although driven by the slow movement of the tectonic plates, occurs regularly on much shorter timescales. Volcanic eruptions replenish the carbon dioxide in the atmosphere, removed by the biosphere, and emit considerable quantities of dust and aerosols. Volcanic activity can therefore affect the energy budget and regulation of the global climate system (Sear et al., 1987).
Number Six, the Human Factor
Another component of the global climate system is an anthropogenic system, mankind. In the last 200 years, through increased utilization of the world’s resources, humans have begun to influence the global climate system, primarily by increasing the Earth’s natural greenhouse effect.
Basic Climate Elements
Temperature is a valuable climate element in climate observation because it directly provides a measure of the energy of the system under inspection.
The measurement of global rainfall offers an indirect or qualitative assessment of the energy of the Earth-atmosphere system. Increased heat storage will increase the rate of evaporation from the oceans (due to higher surface temperatures). In turn, the enhanced levels of water vapor in the atmosphere will intensify global precipitation. Rainfall is, however, subject to significant temporal and spatial variability, and the occurrence of extremes.
Humidity, the amount of water vapor in the air can be described in terms of the water-vapour pressure; the relative humidity; the absolute humidity; the mixing ratio; and the dewpoint. A detailed account of these definitions may be found in Linacre (1992).
Another element is wind, the bulk movement of air of certain strength and direction.
Changes in Climate
Although the climate system is in balance, that balance is dynamic, ever-changing. The system is constantly adjusting to forcing perturbations and, as it adjusts, the climate alters. A change in any one part of the climate system will have much wider consequences as the initial effect cascades through the coupled components of the system. As the effect is transferred from one sub-component of the system to another, it will be modified in character or in scale. In some cases it will be amplified (positive feedback), in others, it may be reduced (negative feedback) (Cess & Potter, 1988).
Additional reading: An Assessment of Climate Feedbacks in Coupled Ocean–Atmosphere Models
Global climate change results from a combination of periodic external and internal forcing mechanisms, and a complex series of interactive feedbacks within the climate system itself.
External forcing sets the pace of climate change; but the internal (non-linear) dynamics of the climate system modulate the final response. This is true for all time scales.
Some of the external forcing mechanisms include galactic variations; orbital variations (factors of the Milankovitch cycles), such as obliquity, eccentricity, precession; and the solar cycle.
The internal mechanisms for climate change include orogeny (the tectonic process of mountain building and continental uplift); epeirogeny (changes in the global disposition of land masses, and like orogenic processes, these changes are driven by internal plate tectonic movements); volcanic activity; ocean circulation; and atmospheric composition.
A longer perspective on climate variability can be obtained by the study of natural phenomena which are climate-dependent. Such phenomena provide a proxy record of the climate.